Translate this page into:
CT-based bone density assessment for iliosacral screw trajectories
Address for correspondence: Dr. Andreas Schicho, Department of Radiology, University of Regensburg, Franz-Josef-Strauß-Allee 11, D-93053 Regensburg, Germany. E-mail: andreas.schicho@googlemail.com
This article was originally published by Wolters Kluwer - Medknow and was migrated to Scientific Scholar after the change of Publisher.
How to cite this article: Schicho A, Gebhard F, Richter PH. CTbased bone density assessment for iliosacral screw trajectories. J Orthop Allied Sci 2016;4:8-13.
Abstract
Introduction:
Sacroiliac screw placement is one standard treatment option for stabilization of posterior pelvic ring injuries encountering high intra- and inter-individual variations of bone stock quality as well as a vast variety and prevalence of sacral dysmorphism. An individual, easy-to-use preoperative bone stock quality estimation would be of high value for the surgeon.
Materials and Methods:
We analyzed 36 standard computed tomography datasets with the uninjured pelvic ring. Using a two-plane cross-referencing technique, we assessed the Hounsfield unit (HU) mean values as well as standard deviation and minimum/maximum values within selected region of interests (ROIs) at five key areas: os ilium left and right, massa lateralis of os sacrum left and right, and central vertebral body on levels S1 and S2.
Results:
Results showed no difference in mean HU at any ROI when comparing male and female data. For all ROIs set on S1 and S2, there was an age-related decline of HU with a calculated slope significantly different from zero. There was no statistical difference of slopes when comparing S1- and S2-level with respect to any distinct ROI. Comparison of levels S1 and S2 revealed differences at the vertebral body and at the right os ilium. The right and left massa lateralis of os sacrum had lower bone density than the center of the vertebral body, the right, or left os ilium on S1; right and left massa lateralis density did not differ significantly. On level S2, results were comparable with no difference of massa lateralis density.
Conclusion:
With our easy-to-use preoperative assessment of bone density of five key areas of sacroiliac screw anchoring we were able to find the lowest bone density in both the left and right massa lateralis on levels S1 and S2 with high inter- and intra-individual variations. Significantly lower bone density was found in the center of the vertebral bodies S2 in comparison to S1, which both are crucial for iliosacral screw placement. We thus recommend priority use of level S1 in screw placement and careful consideration of sole massa lateralis short-screw anchoring.
Keywords
Bone density
computed tomography
Hounsfield units
pelvic ring injury
sacroiliac screw placement
Introduction
Sacroiliac (SI) screw placement is one standard treatment option for stabilization of posterior pelvic ring injuries. Different approaches are used in order to gain maximum stability results, e.g., intraoperative navigation setups,[1] two level (S1 and S2) screw placement,[2] wide span screws traversing the whole os sacrum and both ossa ilia, or polymethyl methacrylate (PMMA) cement augmentation,[3] all addressed by numerous studies. But both biomechanical experiments with cadaver or animal bone as well as computed finite element simulations[4,5] encounter one main problem: High intra- and inter-individual variations of bone stock quality as well as a vast variety and prevalence of sacral dysmorphism[6] limiting the bench-to-bedside transfer of scientific knowledge. An individual, easy-to-use preoperative bone stock quality estimation would be of high value for the surgeon.
Preoperative computed tomography (CT) scans are routinely used to find injuries, identify fracture morphology, and plan screw trajectories[7] while studies[6] suggest "safe zones" for screw placement, identification of individual "stable zones" is needed. One feature of CT imaging is not yet routinely used in daily trauma surgery despite its high informative value concerning bone quality. The Hounsfield unit (HU) is neither an SI-unit nor an SI-derived unit, but it is standardized and reproducible calculated from the CT number, which is an inherent coefficient of CT data acquisition.[8] With respect to some limitations in comparability due to the nature of X-rays, the HU values can be used across different scanner setups, making them a valuable tool as a surrogate descriptor of bone density.[9,10,11] While there is a variety of studies on the use of HU measurements out of the field of oral and maxillofacial surgery,[12,13,14] in trauma surgery first attempts were made recently for opportunistic diagnosis of osteoporosis.[10,15,16]
The objective of the here presented study is to evaluate the individual bone density on both, the S1 and S2 sacroiliac screw trajectories at distinct key areas of biomechanical screw anchoring. Therefore, a fast and easy-to-use CT measurement is used resembling an everyday tool for the surgeon in charge.
Materials and Methods
We enrolled 36 patients, whose standardized polytrauma CT scans were obtained in our emergency department between April 1, 2015, and June 30, 2015, as part of our diagnostic routine according to the scanning protocol outlined below. All subjects were older than 18 years and met both the inclusion (complete CT data set according to our institutions polytrauma scan protocol) and exclusion (incomplete data set; deviations from the standard scan protocol or technique; limitations of data quality, including but not limited to, streak artifacts, motion artifacts, hardening artifacts, presumable photon deprivation due to obesity; fractures, injuries or traumatic alterations to the lumbar spine or pelvis; neoplastic alterations) criteria. Demographic data (date of birth; age) was recorded as included in the CT data set. Inclusion in this study had no effect on patient's treatment or further diagnostics. All human and animal studies have been approved by the ethics committee (no. 183/14) and have therefore been performed in accordance with the ethical standards laid down in the 1964 Declaration of Helsinki and its later amendments.
All CT scans were obtained using a Philips Brilliance 16P CT scanner (Philips GmbH DACH, Hamburg, Germany), 4 mm slice thickness, 120 kV.
The HU scale is a linear transformation of the linear attenuation coefficient measurement into a scale wherein the radiodensity of distilled water (at standard pressure and temperature, [STP]) is defined as 0 (zero) HU, while radiodensity of air (at STP) is defined as - 1000 HU. For a volume X with linear attenuation coefficient μX (and μwater for water and μair for air, respectively), the HU value is calculated as:
Data sets were viewed and analyzed using Agfa Impax EE R20 software (Agfa HealthCare NV, Mortsel, Belgium). In analogy to three-plane-techniques described elsewhere,[10,17] we used a modified two plane cross-referencing technique with transversal and sagittal planes. Average HU values as well as standard deviation, minimum HU, and maximum HU were recorded from region of interests (ROIs) placed as shown in Figure 1 on both levels, S1 and S2, ROIs were placed on the presumable trajectory of an iliosacral screw placement in the transversal planes as described in the literature.[6] In the os ilium on both sides (S1R, S1 L, S2R, S2 L), in the massa lateralis of the os sacrum on both sides (S1RM, S1 LM, S2RM, S2 LM), and central in the vertebral body (S1C, S2C). ROIs were chosen to include a reasonable sized area without covering cancellous bone or edges, osteomes, bone cysts, or other void signals (vessels). Sagittal plane cross-referencing was used for height control of ROI placement, and intra-level variations had to be accepted for simulation of realistic screw placement scenarios.
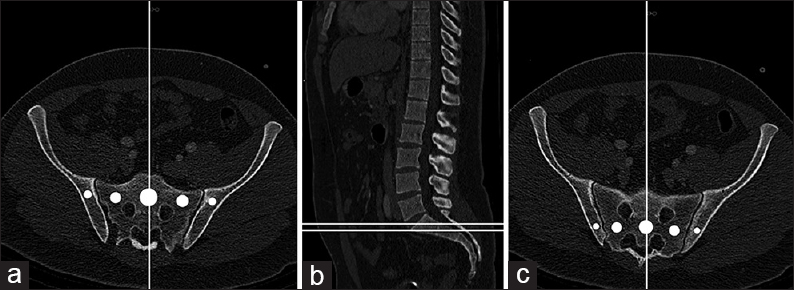
- Cross-referencing and region of interests on level S1 (a) and S2 (c), with corresponding height in the sagittal plane. (b) Key areas from left to right: Right os ilium (R), right massa lateralis of os sacrum (RM), center of vertebral body (c), left massa lateralis of os sacrum (LM), left os ilium (L)
A power analysis with power 0.95 at alpha level 0.05 yielded a minimum sample size of n = 22 for comparison of S1C and S2C.
For statistical calculations, analysis, and plotting, we used GraphPad Prism version 5.00a for Mac (GraphPad Software, San Diego, California, USA). For multiple group analysis, ANOVA with post-hoc Bonferroni comparisons was used. Two groups were compared using two-tailed Student's t-test, paired when suitable. Statistical significant differences were assumed at alpha <0.05.
Results
The mean age of all patients included (n = 36) was 50.39 ± 19.88 years. There was no age difference in the subgroups, male (n = 18; 48.39 ± 20.51 years) and female (n = 18; 52.39 ± 19.61; P = 0.55), respectively. There was no difference in mean HU at any ROI when comparing male and female data (e.g., S1C male 239.4 ± 19.10 HU vs. S1C female 204.0 ± 16.25; P = 0.16; Figure 2).
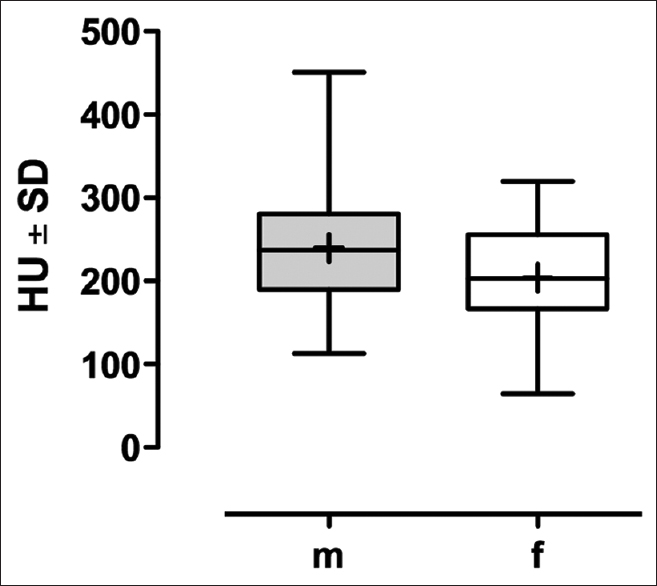
- Influence of gender on vertebral body bone density of S1. No significant difference, data shown in HU ± SD. m = Male, f = Female, HU = Hounsfield unit, SD = Standard deviation
For all ROIs set on S1 and S2, there was an age-related decline of HU with a calculated slope significantly different from zero in all cases [Table 1]. There was no statistical difference of slopes when comparing S1- and S2-level with respect to any distinct ROI. From right to left, slopes on level S1 were 2.875 ± 0.5549 (S1R), −2.124 ± 0.3977 (S1RM), −2.749 ± 0.4592 (S1C), −2.155 ± 0.3536 (S1 LM), and − 2.367 ± 0.5442 (S1 L). On level S2 slopes were − 2.075 ± 0.6258 (S2R), −1.840 ± 0.3722 (S2RM), −2.722 ± 0.5275 (S2C), −1.578 ± 0.4316 (S2 LM), and − 3.215 ± 0.6698 (S2 L). Slopes of age-dependent decline in HU are depicted in Figure 3a for right os ilium, in Figure 3b for right massa lateralis, in Figure 4 for the center of the vertebral body, in [Figure 5a] for the left massa lateralis, and in Figure 5b for the left os ilium. All slopes were significantly different from zero [Table 1].
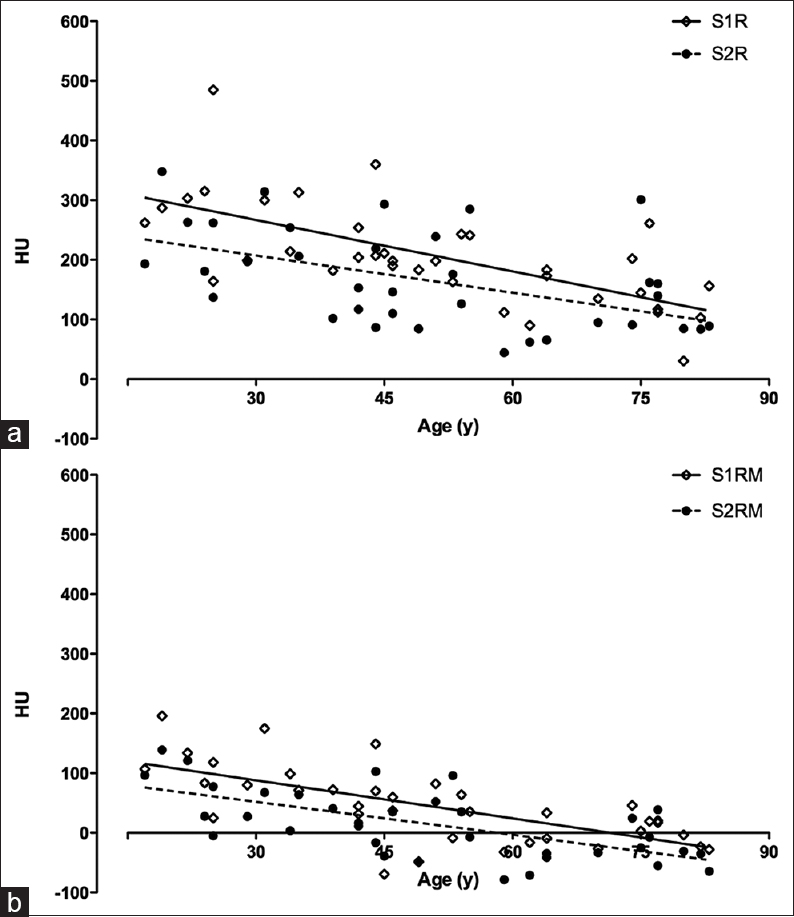
- Decline in mean HU of the right side of the posterior pelvic ring on level S1 (a) and S2 (b). Mean values and fitted linear regression. Data shown are mean HU values, age in years (y). S1R = right os ilium at level S1, S2R = right os ilium at level S2, S1RM = right massa lateralis of os sacrum at level S1, S2RM = right massa lateralis of os sacrum at level S2, HU = Hounsfield unit
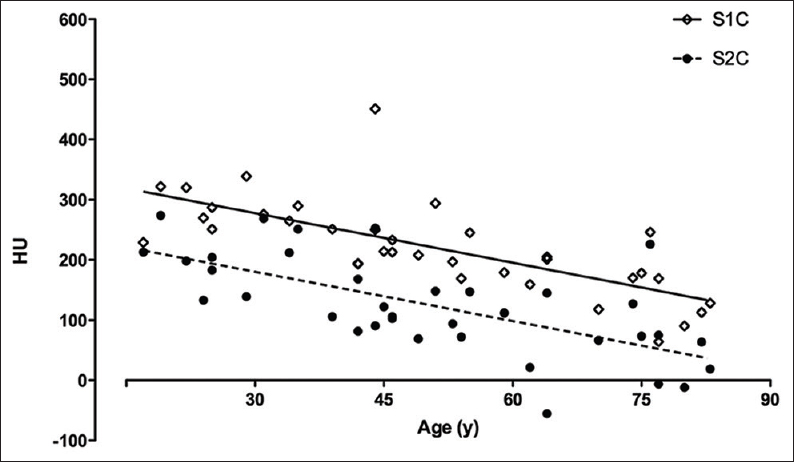
- Decline in mean HU of the vertebral body of S1 (S1C) and S2 (S2C). Mean values (HU) and fitted linear regression. HU = Hounsfield unit
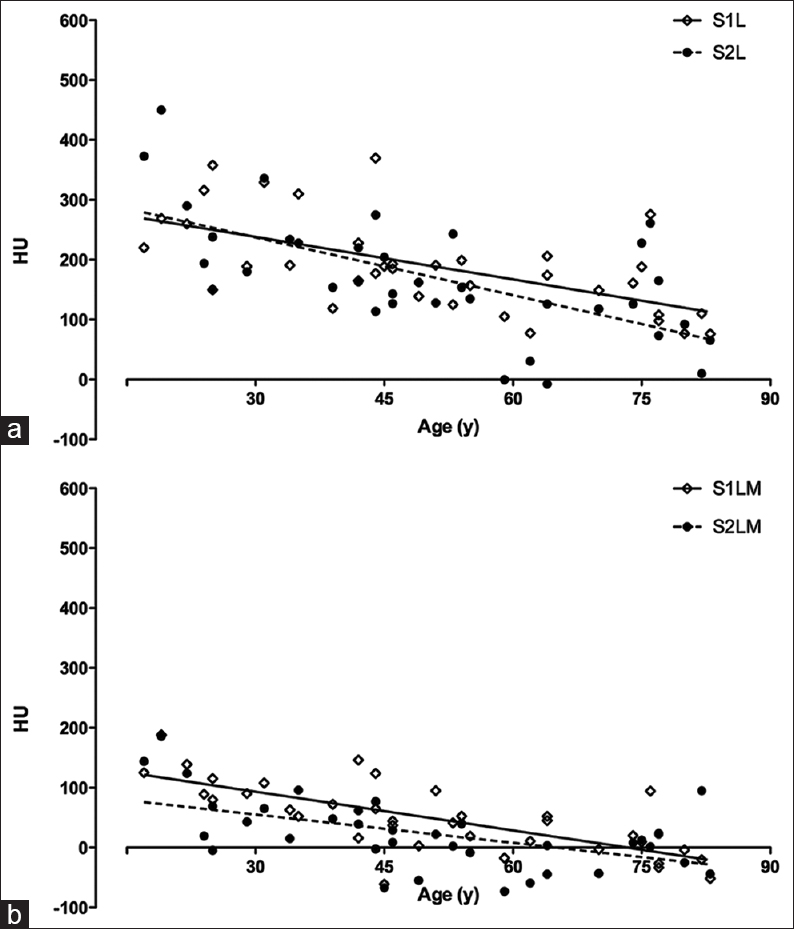
- Decline in mean HU of the left side of the posterior pelvic ring on level S1 (a) and S2 (b). Mean values and fitted linear regression. Data shown are mean HU values, age in years (y). S1 L = left os ilium at level S1. S2 L = left os ilium at level S2. S1 LM = left massa lateralis of os sacrum at level S1. S2 LM = left massa lateralis of os sacrum at level S2, HU = Hounsfield unit
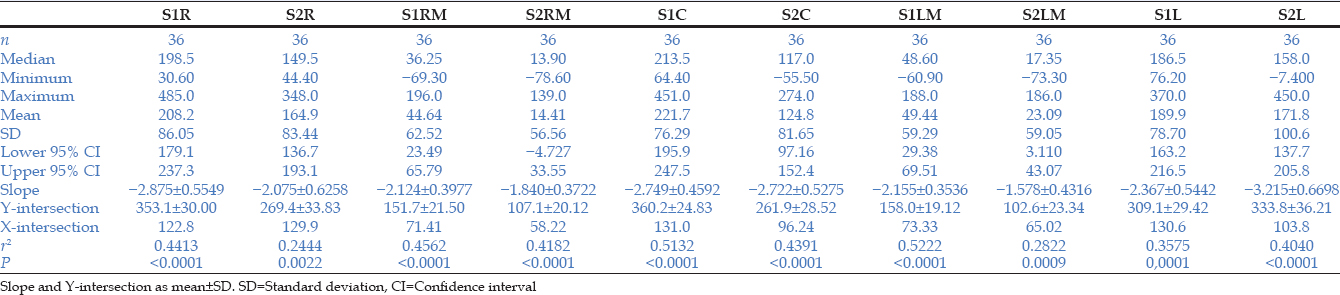
Comparison of levels S1 and S2 revealed differences at the vertebral body (S1C: 221.7 ± 76.29 vs. S2C: 124.8 ± 81.65; P < 0.001; Figure 6) and at the right os ilium (S1R: 208.2 ± 86.05 vs. S2R: 164.9 ± 83.44; P < 0.01; Figure 6). The right (44.64 ± 62.52) and left (49.44 ± 59.29) massa lateralis of os sacrum had lower (P < 0.001) bone density than the center of the vertebral body (221.7 ± 76.29), the right (208.2 ± 86.05), or left (189.9 ± 78.7) os ilium on S1; right and left massa lateralis density did not differ significantly. On level S2, results were comparable with no difference of massa lateralis density (S2RM vs. S2 LM) but both are significantly lower in bone density than S2R, S2C, and S2 L (P < 0.001).
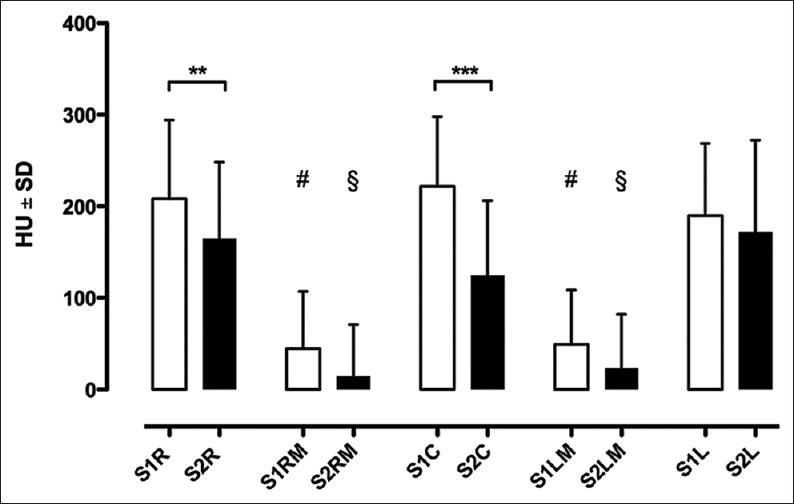
- Comparison of S1 and S2 density at five regions of interests of the posterior pelvic ring. ***P < 0.001, **P < 0.01, #P < 0.001 versus S1R, S1C, and S1 L.§P < 0.001 versus S2R, S2C, and S2L. Data shown are mean HU values ± standard deviation. White for level S1, black for level S2. HU = Hounsfield unit
Discussion
The purpose of the here presented study was to investigate the bone stock quality along both the S1 and S2 screw trajectories to obtain a more detailed knowledge on bone density prior to surgery in order to choose the most stable screw trajectory and avoid implant failure. Therefore, the individually available preoperative CT datasets are used as an easy-to-use tool for surgeon's decision-making and procedure planning.
Our results showed a significant difference in bone density when comparing S1 and S2 vertebral bodies, in conformance with results described elsewhere.[17] In addition, we were able to show a one-sided S1-S2 difference for the right os ilium, but not the left os ilium. This could be due to methodical limitations of the study or due to biomechanistic considerations: The left foot is the dominant foot in up to 85% of the population,[18,19] leading to a mechanical stress-induced preservation of bone density.[20] The massa lateralis on both sides and both levels was identified as the weakest link in the standard iliosacral screw trajectory. A reach of the screw past the low-density massa lateralis into the higher-density vertebral body is expedient, matching the results of finite element simulations.[4,5] Alternatively, perforated screws can be used for massa lateralis PMMA augmentation.[3] The most stable screw placement according to the here presented bone density data is the all-through S1 screw, penetrating both ossa ilia through the vertebral body.
The secondary intention of our study was the descriptive analysis of gender and age influence on bone density of the posterior pelvic ring. There was no significant difference detectable when comparing male and female subgroups; since there was a clear tendency toward females having a lower bone stock density in our cohort, we assume a detectable difference in any larger sized study population. When using linear regression analysis, an almost parallel decline in bone density can be observed for the vertebral bodies of S1 and S2. Although the absolute load to the pelvic ring changes throughout life, the relative load distribution of central S1 and central S2 seems not to. The decline of bone density of the ossa ilia is not parallel, which could be due to one-sided preferable standing, walking, and loading. Still, there is no significant difference in between the left and right os ilium on either level. Although we found a decline in bone density at all key areas using linear regression analysis, r2 values and standard deviations show high inter- and intra-individual variances in bone density. This hints at the value of an individual preoperative assessment of bone quality using the available CT datasets.
With respect to the limitations mentioned below, the bone density measurement in the preoperative data CT yields valuable information for the surgeon. With some data for comparison in mind, the bone quality can be roughly assessed before surgery as low-for-age or high-for-age, giving the chance for preparation of PMMA augmentation equipment or considering two-level screw placement. Measurement of the HU of an ROI does not need repeat measurement, since the built-in tools of state-of-the-art CT imaging software offer mean HU calculations with a display of standard deviation, minimum, and maximum for selected areas. HU measurements are independent of grayscale window width or center,[8] in turn making the often used estimations of bone density from gray scale prone to error.
Besides others,[21] Okuyama et al. reported in spine that the pull-out force correlates with bone density assessed by HU measurement.[22] With a considerably shorter intraosseous screw length, Heller reports the opposite finding for the cervical spine on one hand,[23] but Schreiber reports a positive correlation of bone density and stability in lumbar fusion on the other hand.[24] Reviewing the current literature names the HU bone density measurement a versatile utility for the surgeon that can "alert the treating physician to decreased bone quality, which can be useful in both medically and surgically managing these patients,"[25] encouraging further studies for the clinical implementation of HU measurements.[26]
Limitations of the here presented study and method are: First, in the HU calculation formula, μ depends on the energy of the X-ray photon and differs depending on the material. Thus, the HU depend upon the X-ray energy which depends on the CT scanner set-up. Moreover, for the calculation a monochromatic X-ray spectrum is assumed which, in fact, is not given.[27] But the above-mentioned calculation of HU is acknowledged and accepted as scientific standard. The use of HU measurements as a surrogate marker for bone density is common[10,17] and used as data basis for finite element simulations.[4,5] Second, despite the sample size of the here presented study is statistically sufficient powered, reference data are best based on large cohorts. Thus, the here mentioned HU values would gain in validity with larger sample sizes. Nonetheless, our results and calculations are derived from a wide-based cohort making the given numbers the first reference. Third, the results are based on CT scans of adult patients of the Western European ethnicity only. Since biomechanic and anthropomorphological properties such as body height and weight as well as eating and working habits influence bone density,[28] additional studies are needed to validate the here reported observations for patients of other ethnic backgrounds[29] and for children.
Moreover, the bone density approximation via HU measurement using the already available CT data enables, at no additional cost or radiation exposure, the identification of patients at risk for osteoporosis. Besides the influence on the selection of treatment options in the actual trauma setting, patients can be referred to additional state-of-the-art osteoporosis diagnostics and therapy or preventive measures.
Conclusion
With our easy-to-use preoperative assessment of bone density of five key areas of sacroiliac screw anchoring we were able to find the lowest bone density in both the left and right massa lateralis on levels S1 and S2 with high inter- and intra-individual variations. Significantly lower bone density was found in the center of the vertebral bodies S2 in comparison to S1, which both are crucial for iliosacral screw placement. We thus recommend priority use of level S1 in screw placement and careful consideration of sole massa lateralis short-screw anchoring.
Financial support and sponsorship
Nil.
Conflicts of interest
There are no conflicts of interest.
REFERENCES
- The interdisciplinary hybrid operation theatre. Current experience and future. Chirurg. 2013;84:1036-40.
- [CrossRef] [PubMed] [Google Scholar]
- Biomechanical study of four kinds of percutaneous screw fixation in two types of unilateral sacroiliac joint dislocation: A finite element analysis. Injury. 2014;45:2055-9.
- [CrossRef] [PubMed] [Google Scholar]
- Cement augmentation of the navigated iliosacral screw in the treatment of insufficiency fractures of the sacrum: A new method using modified implants. Int Orthop. 2013;37:1147-50.
- [CrossRef] [PubMed] [Google Scholar]
- Comparison of the risk of breakage of two kinds of sacroiliac screws in the treatment of bilateral sacral fractures. Eur Spine J. 2014;23:1558-67.
- [CrossRef] [PubMed] [Google Scholar]
- Mechanical comparison between lengthened and short sacroiliac screws in sacral fracture fixation: A finite element analysis. Orthop Traumatol Surg Res. 2013;99:601-6.
- [CrossRef] [PubMed] [Google Scholar]
- The role of computed tomography for postoperative evaluation of percutaneous sacroiliac screw fixation and description of a "safe zone" Am J Orthop (Belle Mead NJ). 2014;43:513-6.
- [Google Scholar]
- Standardized posterior pelvic imaging: Use of CT inlet and CT outlet for evaluation and management of pelvic ring injuries. J Orthop Trauma. 2014;28:665-73.
- [CrossRef] [PubMed] [Google Scholar]
- Assessment of osteoporosis using pelvic diagnostic computed tomography. J Bone Miner Metab. 2015;13(8)
- [CrossRef] [PubMed] [Google Scholar]
- Opportunistic osteoporosis screening - Gleaning additional information from diagnostic wrist CT scans. J Bone Joint Surg Am. 2015;97:1095-100.
- [CrossRef] [PubMed] [Google Scholar]
- Use of internal references for assessing CT density measurements of the pelvis as replacement for use of an external phantom. Skeletal Radiol. 2015;44:1597-602.
- [CrossRef] [PubMed] [Google Scholar]
- Assessment of jawbone trabecular bone structure amongst osteoporotic women by cone-beam computed tomography: The OSTEOSYR project. J Investig Clin Dent. 2015;20(4)
- [CrossRef] [PubMed] [Google Scholar]
- Use of dentomaxillofacial cone beam computed tomography in dentistry. World J Radiol. 2015;7:128-30.
- [CrossRef] [PubMed] [Google Scholar]
- Evaluation of bone height and bone mineral density using cone beam computed tomography after secondary bone graft in alveolar cleft. J Craniofac Surg. 2015;26:1463-6.
- [CrossRef] [PubMed] [Google Scholar]
- Osteoporosis in acute fractures of the cervical spine: The role of opportunistic CT screening. J Neurosurg Spine. 2015;23:1-7.
- [CrossRef] [PubMed] [Google Scholar]
- The use of computed tomography attenuation to evaluate osteoporosis following acute fractures of the thoracic and lumbar vertebra. Geriatr Orthop Surg Rehabil. 2014;5:50-5.
- [CrossRef] [PubMed] [Google Scholar]
- Investigation of bone quality of the first and second sacral segments amongst trauma patients: Concerns about iliosacral screw fixation. J Orthop Traumatol. 2015;16:301-8.
- [CrossRef] [PubMed] [Google Scholar]
- Footedness across ages: Distinction between mobilization and stabilization tasks. Laterality. 2015;20:141-53.
- [CrossRef] [PubMed] [Google Scholar]
- The influence of lower-limb dominance on postural balance. Sao Paulo Med J. 2011;129:410-3.
- [CrossRef] [PubMed] [Google Scholar]
- Mechanotransduction and the functional response of bone to mechanical strain. Calcif Tissue Int. 1995;57:344-58.
- [CrossRef] [PubMed] [Google Scholar]
- Biomechanical aspects of primary implant stability: A human cadaver study. Clin Implant Dent Relat Res. 2009;11:113-9.
- [CrossRef] [PubMed] [Google Scholar]
- Stability of transpedicle screwing for the osteoporotic spine. An in vitro study of the mechanical stability. Spine (Phila Pa 1976). 1993;18:2240-5.
- [CrossRef] [PubMed] [Google Scholar]
- Biomechanical study of screws in the lateral masses: Variables affecting pull-out resistance. J Bone Joint Surg Am. 1996;78:1315-21.
- [CrossRef] [PubMed] [Google Scholar]
- An association can be found between Hounsfield units and success of lumbar spine fusion. HSS J. 2014;10:25-9.
- [CrossRef] [PubMed] [Google Scholar]
- Use of computed tomography for assessing bone mineral density. Neurosurg Focus. 2014;37:E4..
- [CrossRef] [PubMed] [Google Scholar]
- Lower preoperative Hounsfield unit measurements are associated with adjacent segment fracture after spinal fusion. Spine (Phila Pa 1976). 2013;38:415-8.
- [CrossRef] [PubMed] [Google Scholar]
- Diagnostic X-ray spectra: A comparison of spectra generated by different computational methods with a measured spectrum. Med Phys. 1998;25:114-20.
- [CrossRef] [PubMed] [Google Scholar]
- Objectively measured physical activity is associated with parameters of bone in 70-year-old men and women. Bone. 2015;81:72-9.
- [CrossRef] [PubMed] [Google Scholar]
- Ethnic differences in bone health. Front Endocrinol (Lausanne). 2015;6:24..
- [CrossRef] [PubMed] [Google Scholar]